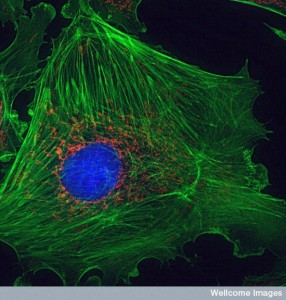
Nature’s Architecture: The cytoskeleton of mammalian cells that allows them to push and pull on their surroundings. Credit: Wellcome Institute via Flickr.
When astronauts escape gravity their bones become weaker, when body-builders lift weights their muscles grow, and when tennis players train their bones become stronger in their hitting arm. These are just three examples of mechanobiology, or how living cells respond to forces.
Cell biologists have paid little attention to engineering in the past, but recent findings suggest that it is time to rethink the role of mechanical forces in biology. Forces are thought to contribute to numerous processes from cancer progression to stem cell differentiation and are likely to be instrumental to treating and preventing a number of debilitating diseases.
Early beginnings
In 1892, Julius Wolff, an orthopaedic surgeon and anatomist, made a novel discovery: bone formation responds to physical loading. He found that fractured bones form an architecture different from that of healthy bones that optimises force transmission following structural damage.
This observation suggests that cells can test their mechanical environment and reorganise their structure in response to changing mechanical conditions. Lacking the necessary tools to investigate this process, forces remained under the radar of the scientific community for nearly a century.
Mechanobiology was further pushed aside during the molecular biology revolution in the 1960s, which set out to explain how genes and proteins contribute to biological function. Researchers focused on developing new tools to better understand biochemical signalling pathways, which describe how chemical signals elicit a physiological response in cells. Following 50 years of research, significant progress has been made, but it is evident that molecules and proteins cannot fully explain these pathways and biologists have begun to reconsider forces.
In 2006, Dennis Discher, a biophysicist and biologist, made an important discovery while studying the effect of forces on stem cell expression. His team placed mesenchymal stem cells, a type of cell that can differentiate into a variety of cell types, on gels of varying stiffness.
Remarkably, they found that cells that are placed on soft gels resembling the stiffness of brain tissue become neurogenic, those placed on medium stiffness gels resembling muscle become myogenic, and those placed on hard gels resembling bone become osteogenic. This finding highlights the significance of the microenvironment on cell differentiation and its implications for tissue disease and regeneration.
How It Works
Cells are situated in the extracellular matrix of tissues and are exposed to a range of external forces. For example, as we walk, our muscles contract, pushing us forward by transmitting forces to our bones through tendons. Our lungs expand as we breathe and our heart pumps oxygen throughout our body by means of blood vessels.
During this relatively simple task, cells in our muscles, tendons, bones, cartilage, lungs, and heart are pushed, pulled, and twisted. These forces are sensed by cells and transmitted into biochemical signals through a process called mechanotransduction, which lies at the heart of mechanobiology.
Recent studies have shown that cells possess a structure called the primary cilium, a rod-like protein that extends from the surface of most mammalian cells. When fluid flows past these structures the rod bends acting as a sensor that can detect fluid flow, similar to a speedometer attached to the bottom of a boat.
When primary cilia bend, their conformational change induces a biochemical response that is translated into changes in biological function. For example, fluid that has a smooth flow has been shown to prevent an inflammatory response in tissue whereas fluid that is turbulent promotes inflammation.
Cells are furthermore capable of producing internal forces that influence tissue development and cell migration. For this reason cells should be viewed as dynamic structures that are constantly pushing and pulling on their microenvironment rather than as static objects like water droplets, whose movement is dictated by external forces.
Recent time-lapse images have captured how skin cells pull on fibres as they migrate through a matrix. When they encounter a weak fibre they let go and attach to another fibre thereby weaving their way through the construct. This observation suggests that skin cells migrate towards areas of high stiffness and are thought to have implications on scar formation and tumour progression, which are much stiffer than healthy tissue.
Looking Ahead
Cell biology was shaped by the development of enabling technologies in the 20th century. Likewise, mechanobiology will rely on the invention of new and sophisticated tools. The recent development of biophysical tools, such as magnetic tweezers, will be key to understanding mechanotransduction mechanisms. Magnetic tweezers are capable of applying piconewton forces to surfaces, that is one trillionth of a newton, and can be used to probe cells to observe their biochemical response.
Although much work is yet to be done in the field of mechanobiology, forces are being used to promote tissue regeneration in the clinic and have shown promising results. For example, over 200,000 amputations have been prevented through the development of a technique called Vacuum Assisted Closure Therapy.
Healing is enhanced by placing a sponge-like material over the wound and applying a cyclic force to the sponge using an oscillating suction pump. Patients undergoing this treatment show enhanced blood vessel generation and cell division due to the micromechanical stimulation of cells.
Forces dominate activities such as breathing and blood flow, but have been shown to play an important role in all cellular functions. Rather than considering forces as a specialised case in biology they should be regarded as an essential part of biological function. With the development of enabling technologies, mechanobiology may dominate the twenty-first century similar to the molecular biology revolution that took place over the past 50 years.